Concretion

A concretion is a hard and compact mass formed by the precipitation of mineral cement within the spaces between particles, and is found in sedimentary rock or soil.[1] Concretions are often ovoid or spherical in shape, although irregular shapes also occur. The word concretion is borrowed from Latin concretio '(act of) compacting, condensing, congealing, uniting', itself derived from concrescere 'to thicken, condense, congeal', from con- 'together' and crescere 'to grow'.[2]
Concretions form within layers of sedimentary strata that have already been deposited. They usually form early in the burial history of the sediment, before the rest of the sediment is hardened into rock. This concretionary cement often makes the concretion harder and more resistant to weathering than the host stratum.
There is an important distinction to draw between concretions and nodules. Concretions are formed from mineral precipitation around some kind of nucleus while a nodule is a replacement body.
Descriptions dating from the 18th century attest to the fact that concretions have long been regarded as geological curiosities. Because of the variety of unusual shapes, sizes and compositions, concretions have been interpreted to be dinosaur eggs, animal and plant fossils (called pseudofossils), extraterrestrial debris or human artifacts.
Origins
[edit]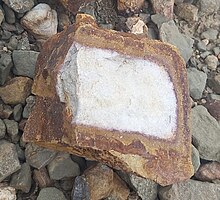
Detailed studies have demonstrated that concretions form after sediments are buried but before the sediment is fully lithified during diagenesis.[3][4][5][6][7][8] They typically form when a mineral precipitates and cements sediment around a nucleus, which is often organic, such as a leaf, tooth, piece of shell or fossil. For this reason, fossil collectors commonly break open concretions in their search for fossil animal and plant specimens.[9] Some of the most unusual concretion nuclei are World War II military shells, bombs, and shrapnel, which are found inside siderite concretions found in an English coastal salt marsh.[10]
Depending on the environmental conditions present at the time of their formation, concretions can be created by either concentric or pervasive growth.[11][12] In concentric growth, the concretion grows as successive layers of mineral precipitate around a central core. This process results in roughly spherical concretions that grow with time. In the case of pervasive growth, cementation of the host sediments, by infilling of its pore space by precipitated minerals, occurs simultaneously throughout the volume of the area, which in time becomes a concretion. Concretions are often exposed at the surface by subsequent erosion that removes the weaker, uncemented material.
Appearance
[edit]
Concretions vary in shape, hardness and size, ranging from objects that require a magnifying lens to be clearly visible[13] to huge bodies three meters in diameter and weighing several thousand pounds.[14] The giant, red concretions occurring in Theodore Roosevelt National Park, in North Dakota, are almost 3 m (9.8 ft) in diameter.[15] Spheroidal concretions, as large as 9 m (30 ft) in diameter, have been found eroding out of the Qasr el Sagha Formation within the Faiyum depression of Egypt.[16] Concretions occur in a wide variety of shapes, including spheres, disks, tubes, and grape-like or soap bubble-like aggregates.[17]
Composition
[edit]
Concretions are commonly composed of a mineral present as a minor component of the host rock. For example, concretions in sandstones or shales are commonly formed of a carbonate mineral such as calcite; those in limestones are commonly an amorphous or microcrystalline form of silica such as chert, flint, or jasper; while those in black shale may be composed of pyrite.[18] Other minerals that form concretions include iron oxides or hydroxides (such as goethite and hematite),[19][20] dolomite, siderite,[21] ankerite,[22] marcasite,[23] barite,[24][25] and gypsum.[26]
Although concretions often consist of a single dominant mineral,[27] other minerals can be present depending on the environmental conditions that created them. For example, carbonate concretions, which form in response to the reduction of sulfates by bacteria, often contain minor percentages of pyrite.[28] Other concretions, which formed as a result of microbial sulfate reduction, consist of a mixture of calcite, barite, and pyrite.[29]
Occurrence
[edit]

Concretions are found in a variety of rocks, but are particularly common in shales, siltstones, and sandstones.[30] They often outwardly resemble fossils or rocks that look as if they do not belong to the stratum in which they were found.[31] Occasionally, concretions contain a fossil, either as its nucleus or as a component that has been incorporated during its growth but concretions are not fossils themselves.[18] They appear in nodular patches, concentrated along bedding planes,[18] or protruding from weathered cliffsides.[32]
Small hematite concretions or Martian spherules have been observed by the Opportunity rover in the Eagle Crater on Mars.[33]
Types of concretions
[edit]Concretions vary considerably in their compositions, shapes, sizes and modes of origin.
Boji Stones are metallic, brownish-gray pyrite/palladium stones, not an actual concretion, but they are indeed round. They usually occur in spherical shapes; on occasion you may see other shapes such as oval or tubular. Some Boji's have a grainy surface resembling sandpaper, (these are the female boji's) while others are studded with protruding pyrite platelets, (the male boji stones). Boji's come in pairs; male and female. This represents Ying/Yang or balance. Boji stones are mainly used in healing work to release blockages and to balance/align the chakras (energy centers). Boji stones are very grounding, allowing you to feel supported and nurtured by the Earth. They are wonderful for relieving arthritis and back pain.
WHY BOJIS® ARE CALLED MALE AND FEMALE
The way that Bojis were first found, there was one crystallized one in the center and 20-50 smooth ones around it. Karen decided that the center one was a chauvinistic and had a harem! The crystallized center stones are found as large as 5 pounds at the most. Females out number males in nature, males are usually heavier in weight, and males are "showier" than females in most species (for example, birds). Therefore, prices are higher for "males" simply because there are less of them. Each Boji has an electromagnetic energy with a negative and a positive side. For purposes of physical energy balancing or healing, normally two stones are paired, one of which is crystallized or "male", the other is which is smooth or "female". The male stone is placed on the weak side of the body (either the side that is damaged, or the left side for right-handed people or vice versa). However, two smooth or two rough stones can also be paired. In this instance, the stone that is heavier should be placed on the weak side.
HOW TO UE BOJI STONES
[edit]Here is an easy way to use the healing energies of Boji stones to balance your energy field: Hold a Boji stone in the fingers of each hand. Now move the stones towards and then aways from each other; you should feel a very subtle pulling and pushing sensation. If you do not feel this, rotate the boji's between your fingers and go through this procedure again. You may feel that the male stone feels better in your right hand rather than your left, or vice a versa. Keep at it until you can feel this pulling/pushing sensation. This allows you to become familiar with the energies of your Boji stones. When you feel the strongest sensation, take notice of which stone is in which hand as well as position. Once you feel you are ready. lay down and place the Boji from your left hand above your head and the other one below your feet. Close your eyes and visualize the golden healing light of the Boji stones as they work to release energy blocks and to balance/align your energy field.
They like to be stored in a natural cloth such as cotton, silk or even leather. I store mine in red silk for the vibration of red energizes the boji stones. You can also place them under a metal type Pyramid or on top of a Reiki grid to further enhance their healing abilities.
Numerology-Boji Stones vibrate to the numbers 1 and 9.
Gemological properties- The Boji Stones are found around the bottom of a natural earth pyramid several stories high. They are uncovered as the earth erodes from them, and are found growing on stems. Geologists say this pyramid is on the heart of the United States. Bojis are a total bleed of fossil and have a hardness of 7.4 on the Moe’s Scale. Unlike any other stone, they are held together by the energy within. Of the energy in a Boji is depleted or destroyed, the stone changes molecule structure from 7.4 to dust. Geologists find that this is a most unusual aspect of the Boji stone. One geologist said the Boji reminded him of the human body that is held together by a life energy when that energy leaves, the rock decays and chemical and mineral elements turn to dust.
© 2001 By Kellie Jo Conn, GG,
Septarian concretions
[edit]

Septarian concretions (or septarian nodules) are carbonate-rich concretions containing angular cavities or cracks (septaria; sg. septarium, from the Latin septum "partition, separating element", referring to the cracks or cavities separating polygonal blocks of hardened material).[34][35] Septarian nodules are characteristically found in carbonate-rich mudrock. They typically show an internal structure of polyhedral blocks (the matrix) separated by mineral-filled radiating cracks (the septaria) which taper towards the rim of the concretion. The radiating cracks sometimes intersect a second set of concentric cracks.[36][34] However, the cracks can be highly variable in shape and volume, as well as the degree of shrinkage they indicate.[37] The matrix is typically composed of argillaceous carbonate, such as clay ironstone, while the crack filling is usually calcite.[36][34] The calcite often contains significant iron (ferroan calcite) and may have inclusions of pyrite and clay minerals. The brown calcite common in septaria may also be colored by organic compounds produced by bacterial decay of organic matter in the original sediments.[38]
Septarian concretions are found in many kinds of mudstone, including lacustrine siltstones such as the Beaufort Group of northwest Mozambique,[39] but are most commonly found in marine shales, such as the Staffin Shale Formation of Skye,[38] the Kimmeridge Clay of England,[40][41] or the Mancos Group of North America.[42]
It is commonly thought that concretions grew incrementally from the inside outwards. Chemical and textural zoning in many concretions are consistent with this concentric model of formation. However, the evidence is ambiguous, and many or most concretions may have formed by pervasive cementation of the entire volume of the concretion at the same time.[43][44][38] For example, if the porosity after early cementation varies across the concretion, then later cementation filling this porosity would produce compositional zoning even with uniform pore water composition.[44] Whether the initial cementation was concentric or pervasive, there is considerable evidence that it occurred quickly and at shallow depth of burial.[45][46][47][38] In many cases, there is clear evidence that the initial concretion formed around some kind of organic nucleus.[48]
The origin of the carbonate-rich septaria is still debated. One possibility is that dehydration hardens the outer shell of the concretion while causing the interior matrix to shrink until it cracks.[36][34] Shrinkage of a still-wet matrix may also take place through syneresis, in which the particles of colloidal material in the interior of the concretion become gradually more tightly bound while expelling water.[39] Another possibility is that early cementation reduces the permeability of the concretion, trapping pore fluids and creating excess pore pressure during continued burial. This could crack the interior at depths as shallow as 10 meters (33 ft).[49] A more speculative theory is that the septaria form by brittle fracturing resulting from earthquakes.[50] Regardless of the mechanism of crack formation, the septaria, like the concretion itself, likely form at a relatively shallow depth of burial of less than 50 meters (160 ft)[51] and possibly as little as 12 meters (39 ft). Geologically young concretions of the Errol Beds of Scotland show texture consistent with formation from flocculated sediments containing organic matter, whose decay left tiny gas bubbles (30 to 35 microns in diameter) and a soap of calcium fatty acids salts. The conversion of these fatty acids to calcium carbonate may have promoted shrinkage and fracture of the matrix.[46][38]
One model for the formation of septarian concretions in the Staffin Shales suggests that the concretions started as semirigid masses of flocculated clay. The individual colloidal clay particles were bound by extracellular polymeric substances or EPS produced by colonizing bacteria. The decay of these substances, together with syneresis of the host mud, produced stresses that fractured the interiors of the concretions while still at shallow burial depth. This was possible only with the bacterial colonization and the right sedimentation rate. Additional fractures formed during subsequent episodes of shallow burial (during the Cretaceous) or uplift (during the Paleogene). Water derived from rain and snow (meteoric water) later infiltrated the beds and deposited ferroan calcite in the cracks.[38]
Septarian concretions often record a complex history of formation that provides geologists with information on early diagenesis, the initial stages of the formation of sedimentary rock from unconsolidated sediments. Most concretions appear to have formed at depths of burial where sulfate-reducing microorganisms are active.[41][52] This corresponds to burial depths of 15 to 150 meters (49 to 492 ft), and is characterized by generation of carbon dioxide, increased alkalinity and precipitation of calcium carbonate.[53] However, there is some evidence that formation continues well into the methanogenic zone beneath the sulfate reduction zone.[54][38][42]
A spectacular example of boulder septarian concretions, which are as much as 3 meters (9.8 feet) in diameter, are the Moeraki Boulders. These concretions are found eroding out of Paleocene mudstone of the Moeraki Formation exposed along the coast near Moeraki, South Island, New Zealand. They are composed of calcite-cemented mud with septarian veins of calcite and rare late-stage quartz and ferrous dolomite.[55][56][57][58] The much smaller septarian concretions found in the Kimmeridge Clay exposed in cliffs along the Wessex coast of England are more typical examples of septarian concretions.[59]
Cannonball concretions
[edit]
Cannonball concretions are large spherical concretions, which resemble cannonballs. These are found along the Cannonball River within Morton and Sioux Counties, North Dakota, and can reach 3 m (9.8 ft) in diameter. They were created by early cementation of sand and silt by calcite. Similar cannonball concretions, which are as much as 4 to 6 m (13 to 20 ft) in diameter, are found associated with sandstone outcrops of the Frontier Formation in northeast Utah and central Wyoming. They formed by the early cementation of sand by calcite.[60] Somewhat weathered and eroded giant cannonball concretions, as large as 6 meters (20 feet) in diameter, occur in abundance at "Rock City" in Ottawa County, Kansas. Large and spherical boulders are also found along Koekohe beach near Moeraki on the east coast of the South Island of New Zealand.[61] The Moeraki Boulders, Ward Beach boulders and Koutu Boulders of New Zealand are examples of septarian concretions, which are also cannonball concretions. Large spherical rocks, which are found on the shore of Lake Huron near Kettle Point, Ontario, and locally known as "kettles", are typical cannonball concretions. Cannonball concretions have also been reported from Van Mijenfjorden, Spitsbergen; near Haines Junction, Yukon Territory, Canada; Jameson Land, East Greenland; near Mecevici, Ozimici, and Zavidovici in Bosnia-Herzegovina; in Alaska in the Kenai Peninsula Captain Cook State Park on north of Cook Inlet beach[62] and on Kodiak Island northeast of Fossil Beach.[63] This type of concretion is also found in Romania, where they are known as trovants.[64][65]
Hiatus concretions
[edit]

Hiatus concretions are distinguished by their stratigraphic history of exhumation, exposure and reburial. They are found where submarine erosion has concentrated early diagenetic concretions as lag surfaces by washing away surrounding fine-grained sediments.[66] Their significance for stratigraphy, sedimentology and paleontology was first noted by Voigt who referred to them as Hiatus-Konkretionen.[67] "Hiatus" refers to the break in sedimentation that allowed this erosion and exposure. They are found throughout the fossil record but are most common during periods in which calcite sea conditions prevailed, such as the Ordovician, Jurassic and Cretaceous.[66] Most are formed from the cemented infillings of burrow systems in siliciclastic or carbonate sediments.
A distinctive feature of hiatus concretions separating them from other types is that they were often encrusted by marine organisms including bryozoans, echinoderms and tube worms in the Paleozoic[68] and bryozoans, oysters and tube worms in the Mesozoic and Cenozoic. Hiatus concretions are also often significantly bored by worms and bivalves.[69]
Elongate concretions
[edit]Elongate concretions form parallel to sedimentary strata and have been studied extensively due to the inferred influence of phreatic (saturated) zone groundwater flow direction on the orientation of the axis of elongation.[70][60][71][72] In addition to providing information about the orientation of past fluid flow in the host rock, elongate concretions can provide insight into local permeability trends (i.e., permeability correlation structure; variation in groundwater velocity,[73] and the types of geological features that influence flow.
Elongate concretions are well known in the Kimmeridge Clay formation of northwest Europe. In outcrops, where they have acquired the name "doggers", they are typically only a few meters across, but in the subsurface they can be seen to penetrate up to tens of meters of along-hole dimension. Unlike limestone beds, however, it is impossible to consistently correlate them between even closely spaced wells.[citation needed]
Moqui Marbles
[edit]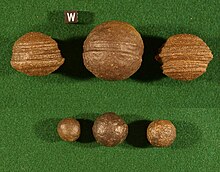
Moqui Marbles, also called Moqui balls or "Moki marbles", are iron oxide concretions which can be found eroding in great abundance out of outcrops of the Navajo Sandstone within south-central and southeastern Utah. These concretions range in shape from spheres to discs, buttons, spiked balls, cylindrical forms, and other odd shapes. They range from pea-size to baseball-size.[74][75]
The concretions were created by the precipitation of iron, which was dissolved in groundwater. The iron was originally present as a thin film of iron oxide surrounding sand grains in the Navajo Sandstone. Groundwater containing methane or petroleum from underlying rock beds reacted with the iron oxide, converting it to soluble reduced iron. When the iron-bearing groundwater came into contact with more oxygen-rich groundwater, the reduced iron was converted back to insoluble iron oxide, which formed the concretions.[74][75][76] It is possible that reduced iron first formed siderite concretions that were subsequently oxidized. Iron-oxidizing bacteria may have played a role.[77]
Kansas pop rocks
[edit]Kansas pop rocks are concretions of either iron sulfide, i.e. pyrite and marcasite, or in some cases jarosite, which are found in outcrops of the Smoky Hill Chalk Member of the Niobrara Formation within Gove County, Kansas. They are typically associated with thin layers of altered volcanic ash, called bentonite, that occur within the chalk comprising the Smoky Hill Chalk Member. A few of these concretions enclose, at least in part, large flattened valves of inoceramid bivalves. These concretions range in size from a few millimeters to as much as 0.7 m (2.3 ft) in length and 12 cm (0.39 ft) in thickness. Most of these concretions are oblate spheroids. Other "pop rocks" are small polycuboid pyrite concretions, which are as much as 7 cm (0.23 ft) in diameter. These concretions are called "pop rocks" because they explode if thrown in a fire. Also, when they are either cut or hammered, they produce sparks and a burning sulfur smell. Contrary to what has been published on the Internet, none of the iron sulfide concretions, which are found in the Smoky Hill Chalk Member were created by either the replacement of fossils or by metamorphic processes. In fact, metamorphic rocks are completely absent from the Smoky Hill Chalk Member.[78] Instead, all of these iron sulfide concretions were created by the precipitation of iron sulfides within anoxic marine calcareous ooze after it had accumulated and before it had lithified into chalk.
Iron sulfide concretions, such as the Kansas Pop rocks, consisting of either pyrite and marcasite, are nonmagnetic.[79] On the other hand, iron sulfide concretions, which either are composed of or contain either pyrrhotite or smythite, will be magnetic to varying degrees.[80] Prolonged heating of either a pyrite or marcasite concretion will convert portions of either mineral into pyrrhotite causing the concretion to become slightly magnetic.
Claystones, clay dogs, and fairy stones
[edit]Disc concretions composed of calcium carbonate are often found eroding out of exposures of interlaminated silt and clay, varved, proglacial lake deposits. For example, great numbers of strikingly symmetrical concretions have been found eroding out of outcrops of Quaternary proglacial lake sediments along and in the gravels of the Connecticut River and its tributaries in Massachusetts and Vermont. Depending the specific source of these concretions, they vary in an infinite variety of forms that include disc-shapes; crescent-shapes; watch-shapes; cylindrical or club-shapes; botryoidal masses; and animal-like forms. They can vary in length from 2 in (5.1 cm) to over 22 in (56 cm) and often exhibit concentric grooves on their surfaces. In the Connecticut River Valley, these concretions are often called "claystones" because the concretions are harder than the clay enclosing them. In local brickyards, they were called "clay-dogs" either because of their animal-like forms or the concretions were nuisances in molding bricks.[81][82][83] Similar disc-shaped calcium carbonate concretions have also been found in the Harricana River valley in the Abitibi-Témiscamingue administrative region of Quebec, and in Östergötland county, Sweden. In Scandinavia, they are known as "marlekor" ("fairy stones").[84][85]
Gogottes
[edit]
Gogottes are sandstone concretions found in Oligocene (~30 million years) aged sediments near Fontainebleau, France. Gogottes have fetched high prices at auction due to their sculpture-like quality.[86]
See also
[edit]- Bowling Ball Beach – Beach in Mendocino County, California, US
- Caliche, also known as calcrete – Calcium carbonate based concretion of sediment in arid and semi-arid soils
- Champ Island – Island in Franz Josef Land, Russia
- Diagenesis – Physico-chemical changes in sediments occurring after their deposition
- Dinocochlea – Trace fossil in the Natural History Museum, London
- Dorodango – Japanese art form in which earth and water are molded to create a delicate shiny sphere
- Gypcrust – Hardened layer of soil with a high percentage of gypsum. CaSO4 concretions in arid and semi-arid soils
- Klerksdorp sphere – Natural nodule-like rock concretions
- Martian spherules – Small iron oxide spherules found on Mars
- Moeraki Boulders – Large spherical boulders on Otago coast, New Zealand
- Mushroom Rock State Park – State park in Kansas, United States
- Nodule (geology) – Small mass of a mineral with a contrasting composition to the enclosing sediment or rock, a replacement body, not to be confused with a concretion
- Rock City, Kansas – park in Kansas, United States of America, United States of America
- Speleothem – Structure formed in a cave by the deposition of minerals from water. CaCO3
References
[edit]- ^ Glossary of terms in soil science (PDF). Ottawa: Agriculture Canada. 1976. p. 13. ISBN 0662015339.
- ^ Harper, Douglas. "concretion". Online Etymology Dictionary.
- ^ Dale, P.; Landis, C. A.; Boles, J. R. (1985-05-01). "The Moeraki Boulders; anatomy of some septarian concretions". Journal of Sedimentary Research. 55 (3): 398–406. doi:10.1306/212F86E3-2B24-11D7-8648000102C1865D. ISSN 1527-1404.
- ^ Boles, James R.; Thyne, Geoffrey D. (1989-03-01). "Isotopic evidence for origin of the Moeraki septarian concretions, New Zealand". Journal of Sedimentary Research. 59 (2): 272–279. doi:10.1306/212F8F6C-2B24-11D7-8648000102C1865D. ISSN 1527-1404.
- ^ Scotchman, I. C. (1991). "The geochemistry of concretions from the Kimmeridge Clay Formation of southern and eastern England". Sedimentology. 38 (1): 79–106. Bibcode:1991Sedim..38...79S. doi:10.1111/j.1365-3091.1991.tb01856.x. ISSN 1365-3091.
- ^ Mozley, Peter S.; Burns, Stephen J. (1993). "Oxygen and Carbon Isotopic Composition of Marine Carbonate Concretions: An Overview". SEPM Journal of Sedimentary Research. 63. doi:10.1306/D4267A91-2B26-11D7-8648000102C1865D. Retrieved 19 August 2021.
- ^ Milliken, Kitty L.; Picard, M. Dane; McBride, Earle F. (2003-05-01). "Calcite-Cemented Concretions in Cretaceous Sandstone, Wyoming and Utah, U.S.A." Journal of Sedimentary Research. 73 (3): 462–483. Bibcode:2003JSedR..73..462M. doi:10.1306/111602730462. ISSN 1527-1404.
- ^ Davis, J. Matthew; Mozley, Peter S. (2005-11-01). "Internal structure and mode of growth of elongate calcite concretions: Evidence for small-scale, microbially induced, chemical heterogeneity in groundwater". GSA Bulletin. 117 (11–12): 1400–1412. Bibcode:2005GSAB..117.1400M. doi:10.1130/B25618.1. ISSN 0016-7606.
- ^ Prothero, Donald R.; Schwab, Fred (2004). Sedimentary geology : an introduction to sedimentary rocks and stratigraphy (2nd ed.). New York: W.H. Freeman. p. 118. ISBN 0716739054.
- ^ Esson, J.; Curtis, C. D.; Burley, S. D.; Al-AGHA, M. R. (1995-02-01). "Complex cementation textures and authigenic mineral assemblages in Recent concretions from the Lincolnshire Wash (east coast, UK) driven by Fe(0) to Fe(II) oxidation" (PDF). Journal of the Geological Society. 152 (1): 157–171. Bibcode:1995JGSoc.152..157A. doi:10.1144/gsjgs.152.1.0157. ISSN 0016-7649. S2CID 129359274. Archived from the original (PDF) on 2019-12-13.
- ^ Davis, J. Matthew; Mozley, Peter S. (1996-01-01). "Relationship between oriented calcite concretions and permeability correlation structure in an alluvial aquifer, Sierra Ladrones Formation, New Mexico". Journal of Sedimentary Research. 66 (1): 11–16. doi:10.1306/D4268293-2B26-11D7-8648000102C1865D. ISSN 1527-1404.
- ^ Fisher, Q. J.; Raiswell, R. (2000-01-01). "Mudrock-hosted carbonate concretions: a review of growth mechanisms and their influence on chemical and isotopic composition". Journal of the Geological Society. 157 (1): 239–251. Bibcode:2000JGSoc.157..239R. doi:10.1144/jgs.157.1.239. ISSN 0016-7649. S2CID 128897857.
- ^ Allaby, Michael (2013). "concretion". A dictionary of geology and earth sciences (Fourth ed.). Oxford: Oxford University Press. ISBN 9780199653065.
- ^ Boggs, Sam (2006). Principles of sedimentology and stratigraphy (4th ed.). Upper Saddle River, N.J.: Pearson Prentice Hall. p. 114. ISBN 0131547283.
- ^ "Theodore Roosevelt National Park North Unit Scenic Byway". Archived from the original on 2012-05-08. Retrieved 2012-05-13.
- ^ Abdel-Wahab, A.; McBride, E. F. (1 January 2001). "Origin of Giant Calcite-Cemented Concretions, Temple Member, Qasr El Sagha Formation (Eocene), Faiyum Depression, Egypt". Journal of Sedimentary Research. 71 (1): 70–81. Bibcode:2001JSedR..71...70A. doi:10.1306/031700710070.
- ^ Todd, J. E. (1 January 1903). "Concretions and their geological effects". Geological Society of America Bulletin. 14 (1): 353–368. Bibcode:1903GSAB...14..353T. doi:10.1130/GSAB-14-353.
- ^ a b c Prothero & Schwab 2004, p. 118.
- ^ Busigny, Vincent; Dauphas, Nicolas (February 2007). "Tracing paleofluid circulations using iron isotopes: A study of hematite and goethite concretions from the Navajo Sandstone (Utah, USA)". Earth and Planetary Science Letters. 254 (3–4): 272–287. Bibcode:2007E&PSL.254..272B. doi:10.1016/j.epsl.2006.11.038.
- ^ Parry, W.T. (January 2011). "Composition, nucleation, and growth of iron oxide concretions". Sedimentary Geology. 233 (1–4): 53–68. Bibcode:2011SedG..233...53P. doi:10.1016/j.sedgeo.2010.10.009.
- ^ Curtis, C.D.; Coleman, M.L.; Love, L.G. (October 1986). "Pore water evolution during sediment burial from isotopic and mineral chemistry of calcite, dolomite and siderite concretions". Geochimica et Cosmochimica Acta. 50 (10): 2321–2334. Bibcode:1986GeCoA..50.2321C. doi:10.1016/0016-7037(86)90085-2.
- ^ Strickler, Michael E. (1990). "Fe Substitution for Al in Glauconite with Increasing Diagenesis in the First Wilcox Sandstone (Lower Eocene), Livingston Parish, Louisiana". Clays and Clay Minerals. 38 (1): 69–76. Bibcode:1990CCM....38...69S. doi:10.1346/CCMN.1990.0380110. S2CID 140180525.
- ^ Van Horn, F.R.; Van Horn, K.R. (1933). "X-ray study of pyrite or marcasite concretions in the rocks of the Cleveland, Ohio, quadrangles". American Mineralogist. 18 (7): 288–294. Retrieved 10 August 2021.
- ^ Bréhéret, Jean-G.; Brumsack, Hans-J. (February 2000). "Barite concretions as evidence of pauses in sedimentation in the Marnes Bleues Formation of the Vocontian Basin (SE France)". Sedimentary Geology. 130 (3–4): 205–228. Bibcode:2000SedG..130..205B. doi:10.1016/S0037-0738(99)00112-8.
- ^ Leśniak, P.M.; Łącka, B.; Hladı́kova, J.; Zieliński, G. (June 1999). "Origin of barite concretions in the West Carpathian flysch, Poland". Chemical Geology. 158 (1–2): 155–163. Bibcode:1999ChGeo.158..155L. doi:10.1016/S0009-2541(99)00010-8.
- ^ Cruz, Marí Pí, A (2015). "Characterization of Gypsum Concretion in Loess: Some Geotechnical Considerations". From Fundamentals to Applications in Geotechnics (From Fundamentals to Applications in Geotechnics): 3248–3255. doi:10.3233/978-1-61499-603-3-3248.
{{cite journal}}
: CS1 maint: numeric names: authors list (link) - ^ Allaby 2013, "concretion".
- ^ Coleman, Max L. (July 1993). "Microbial processes: Controls on the shape and composition of carbonate concretions". Marine Geology. 113 (1–2): 127–140. Bibcode:1993MGeol.113..127C. doi:10.1016/0025-3227(93)90154-N.
- ^ Raiswell, R.; Bottrell, S. H.; Dean, S. P.; Marshall, J. D.; Carr, A.; Hatfield, D. (25 April 2002). "Isotopic constraints on growth conditions of multiphase calcite-pyrite-barite concretions in Carboniferous mudstones: Diagenetic history of septarian concretions in Carboniferous mudstones". Sedimentology. 49 (2): 237–254. doi:10.1046/j.1365-3091.2002.00439.x. S2CID 129664903.
- ^ Boggs 2006, p. 114.
- ^ "Concretions". Paleontological Research Institution. Retrieved 11 August 2021.
- ^ Marshall, Jim D.; Pirrie, Duncan (March 2013). "Carbonate concretions-explained". Geology Today. 29 (2): 53–62. Bibcode:2013GeolT..29...53M. doi:10.1111/gto.12002. S2CID 129659655.
- ^ Dvorsky, George (15 February 2019). "The Enduring Mystery of the Martian 'Blueberries' Discovered by Opportunity Rover". Gizmodo.
- ^ a b c d Jackson, Julia A., ed. (1997). "septarium". Glossary of geology (Fourth ed.). Alexandria, Virginia: American Geological Institute. ISBN 0922152349.
- ^ "septarian". dictionary.reference.com. Retrieved March 20, 2014.
- ^ a b c Potter, Paul Edwin; Maynard, J. Barry; Pryor, Wayne A. (1980). Sedimentology of shale: study guide and reference source. New York: Springer-Verlag. pp. 23, 36. ISBN 0387904301.
- ^ Pratt, Brian R. (27 February 2001). "Septarian concretions: internal cracking caused by synsedimentary earthquakes". Sedimentology. 48 (1): 189, 193–194. Bibcode:2001Sedim..48..189P. doi:10.1046/j.1365-3091.2001.00366.x. S2CID 140665532.
- ^ a b c d e f g Hendry, James P.; Pearson, Michael J.; Trewin, Nigel H.; Fallick, Anthony E. (16 May 2006). "Jurassic septarian concretions from NW Scotland record interdependent bacterial, physical and chemical processes of marine mudrock diagenesis: Jurassic septarian concretions, NW Scotland". Sedimentology. 53 (3): 537–565. doi:10.1111/j.1365-3091.2006.00779.x. S2CID 130767202.
- ^ a b Melezhik, Victor A.; Fallick, Anthony E.; Smith, Richard A.; Rosse, Danta M. (December 2007). "Spherical and columnar, septarian, 18 O-depleted, calcite concretions from Middle–Upper Permian lacustrine siltstones in northern Mozambique: evidence for very early diagenesis and multiple fluids". Sedimentology. 54 (6): 1389–1416. Bibcode:2007Sedim..54.1389M. doi:10.1111/j.1365-3091.2007.00886.x. S2CID 129030770.
- ^ Astin, T. R.; Scotchman, I. C. (April 1988). "The diagenetic history of some septarian concretions from the Kimmeridge Clay, England". Sedimentology. 35 (2): 349–368. Bibcode:1988Sedim..35..349A. doi:10.1111/j.1365-3091.1988.tb00952.x.
- ^ a b Scotchman, I. C. (February 1991). "The geochemistry of concretions from the Kimmeridge Clay Formation of southern and eastern England". Sedimentology. 38 (1): 79–106. Bibcode:1991Sedim..38...79S. doi:10.1111/j.1365-3091.1991.tb01856.x.
- ^ a b Dale, Annabel; John, Cédric M.; Mozley, Peter S.; Smalley, P. C.; Muggeridge, Ann H. (May 2014). "Time-capsule concretions: Unlocking burial diagenetic processes in the Mancos Shale using carbonate clumped isotopes". Earth and Planetary Science Letters. 394: 30–37. Bibcode:2014E&PSL.394...30D. doi:10.1016/j.epsl.2014.03.004.
- ^ Mozley, Peter S. (May 1996). "The internal structure of carbonate concretions in mudrocks: a critical evaluation of the conventional concentric model of concretion growth". Sedimentary Geology. 103 (1–2): 85–91. Bibcode:1996SedG..103...85M. doi:10.1016/0037-0738(95)00087-9.
- ^ a b Raiswell, R.; Fisher, Q. J. (January 2000). "Mudrock-hosted carbonate concretions: a review of growth mechanisms and their influence on chemical and isotopic composition". Journal of the Geological Society. 157 (1): 239–251. Bibcode:2000JGSoc.157..239R. doi:10.1144/jgs.157.1.239. S2CID 128897857.
- ^ Thyne, Geoffrey D.; Boles, James R. (1989). "Isotopic Evidence for Origin of the Moeraki Septarian Concretions, New Zealand". SEPM Journal of Sedimentary Research. 59. doi:10.1306/212F8F6C-2B24-11D7-8648000102C1865D.
- ^ a b Duck, R. W. (February 1995). "Subaqueous shrinkage cracks and early sediment fabrics preserved in Pleistocene calcareous concretions". Journal of the Geological Society. 152 (1): 151–156. Bibcode:1995JGSoc.152..151D. doi:10.1144/gsjgs.152.1.0151. S2CID 129928697.
- ^ De Craen, M.; Swennen, R.; Keppens, E. (1998). "Petrography and geochemistry of septarian carbonate concretions from the Boom Clay Formation (Oligocene, Belgium)". Geologie en Mijnbouw. 77 (1): 63–76. doi:10.1023/A:1003468328212. S2CID 126635562.
- ^ Potter, Maynard & Pryor 1980, p. 23.
- ^ Hounslow, Mark W. (November 1997). "Significance of localized pore pressures to the genesis of septarian concretions". Sedimentology. 44 (6): 1133–1147. Bibcode:1997Sedim..44.1133H. doi:10.1046/j.1365-3091.1997.d01-64.x. S2CID 130385560.
- ^ Pratt 2001, pp. 189–213.
- ^ Astin, T. R. (October 1986). "Septarian crack formation in carbonate concretions from shales and mudstones". Clay Minerals. 21 (4): 617–631. Bibcode:1986ClMin..21..617A. doi:10.1180/claymin.1986.021.4.12. S2CID 128609480.
- ^ Pearson, M.J.; Hendry, J.P.; Taylor, C.W.; Russell, M.A. (April 2005). "Fatty acids in sparry calcite fracture fills and microsparite cement of septarian diagenetic concretions". Geochimica et Cosmochimica Acta. 69 (7): 1773–1786. Bibcode:2005GeCoA..69.1773P. doi:10.1016/j.gca.2004.09.024.
- ^ Raiswell, R.; Fisher, Q.J. (November 2004). "Rates of carbonate cementation associated with sulphate reduction in DSDP/ODP sediments: implications for the formation of concretions" (PDF). Chemical Geology. 211 (1–2): 71–85. Bibcode:2004ChGeo.211...71R. doi:10.1016/j.chemgeo.2004.06.020. Archived from the original (PDF) on 2022-01-30. Retrieved 2021-08-19.
- ^ Huggett, J. M. (October 1994). "Diagenesis of mudrocks and concretions from the London Clay Formation in the London Basin". Clay Minerals. 29 (4): 693–707. Bibcode:1994ClMin..29..693H. doi:10.1180/claymin.1994.029.4.22. S2CID 129727119.
- ^ Boles, J.R.; Landis, C.A.; Dale, P. (1985). "The Moeraki Boulders – Anatomy of Some Septarian Concretions". SEPM Journal of Sedimentary Research. 55: 398–406. doi:10.1306/212F86E3-2B24-11D7-8648000102C1865D.
- ^ Fordyce, E., and P. Maxwell, 2003, Canterbury Basin Paleontology and Stratigraphy, Geological Society of New Zealand Annual Field Conference 2003 Field Trip 8, Miscellaneous Publication 116B, Geological Society of New Zealand, Dunedin, New Zealand. ISBN 0-908678-97-5
- ^ Forsyth, P.J., and G. Coates, 1992, The Moeraki boulders. Institute of Geological & Nuclear Sciences, Information Series no. 1, (Lower Hutt, New Zealand)
- ^ Thyne, G.D., and J.R. Boles, 1989, Isotopic evidence for origin of the Moeraki septarian concretions, New Zealand, Journal of Sedimentary Petrology. v. 59, n. 2, p. 272–279.
- ^ Astin, T. R. (1988). "The diagenetic history of some septarian concretions from the Kimmeridge Clay, England". Sedimentology. 35 (2): 349–368. Bibcode:1988Sedim..35..349A. doi:10.1111/j.1365-3091.1988.tb00952.x.
- ^ a b McBride, E. F.; Picard, M. D.; Milliken, K. L. (1 May 2003). "Calcite-Cemented Concretions in Cretaceous Sandstone, Wyoming and Utah, U.S.A.". Journal of Sedimentary Research. 73 (3): 462–483. Bibcode:2003JSedR..73..462M. doi:10.1306/111602730462.
- ^ Dann, C., and Peat, N. (1989) Dunedin, North and South Otago. Wellington: GP Books. ISBN 0-477-01438-0
- ^ "Kenai Peninsula Online – Alaska Newspaper –". Archived from the original on 2011-07-08. Retrieved 2010-05-13.
- ^ "Geological Survey Professional Paper". U.S. Government Printing Office. 24 May 1976 – via Google Books.
- ^ "trovant". dexonline.ro. Retrieved October 3, 2024.
- ^ Emma Davies, "These ‘living’ rocks can give birth to baby stones", 8 August 2023, BBC Science Focus
- ^ a b Zatoń, Michał (24 September 2010). "Hiatus concretions". Geology Today. 26 (5): 186–189. Bibcode:2010GeolT..26..186Z. doi:10.1111/j.1365-2451.2010.00762.x. S2CID 247665440.
- ^ Voigt, Ehrhard (October 1968). "Über Hiatus-Konkretionen (dargestellt an Beispielen aus dem Lias)". Geologische Rundschau. 58 (1): 281–296. Bibcode:1968GeoRu..58..281V. doi:10.1007/BF01820609. S2CID 128842746.
- ^ Wilson, M. A. (3 May 1985). "Disturbance and Ecologic Succession in an Upper Ordovician Cobble-Dwelling Hardground Fauna". Science. 228 (4699): 575–577. Bibcode:1985Sci...228..575W. doi:10.1126/science.228.4699.575. PMID 17736081. S2CID 28818298.
- ^ Wilson, Mark A.; Taylor, Paul D. (February 2001). "Palaeocology of Hard Substrate Faunas from the Cretaceous Qahlah Formation of the Oman Mountains". Palaeontology. 44 (1): 21–41. Bibcode:2001Palgy..44...21W. doi:10.1111/1475-4983.00167. S2CID 129664357.
- ^ Johnson, M.R. (1989). "Paleogeographic Significance of Oriented Calcareous Concretions in the Triassic Katberg Formation, South Africa". SEPM Journal of Sedimentary Research. 59: 1008–1010. doi:10.1306/212F90D9-2B24-11D7-8648000102C1865D.
- ^ Mozley, Peter S.; Goodwin, Laurel B. (1 June 1995). "Patterns of cementation along a Cenozoic normal fault: A record of paleoflow orientations". Geology. 23 (6): 539–542. Bibcode:1995Geo....23..539M. doi:10.1130/0091-7613(1995)023<0539:POCAAC>2.3.CO;2.
- ^ Mozley, Peter S.; Davis, J. Matthew (2005). "Internal structure and mode of growth of elongate calcite concretions: Evidence for small-scale, microbially induced, chemical heterogeneity in groundwater". Geological Society of America Bulletin. 117 (11): 1400. Bibcode:2005GSAB..117.1400M. doi:10.1130/B25618.1.
- ^ Davis, J. Matthew (June 1999). "Oriented carbonate concretions in a paleoaquifer: Insights into geologic controls on fluid flow". Water Resources Research. 35 (6): 1705–1711. Bibcode:1999WRR....35.1705D. doi:10.1029/1999WR900042. S2CID 129502157.
- ^ a b Chan, M.A.; Parry, W.T. (2002). "Mysteries of Sandstone Colors and Concretions in Colorado Plateau Canyon Country" (PDF). Utah Geological Survey Public Information Series. 77: 1–19. Retrieved 18 August 2021.
- ^ a b Catling, David C. (June 2004). "On Earth, as it is on Mars?". Nature. 429 (6993): 707–708. doi:10.1038/429707a. PMID 15201892. S2CID 4393420.
- ^ Chan, M.A.; Beitler, B.B.; Parry, W.T.; Ormo, J.; Komatsu, G. (2005). "Red Rock and Red Planet Diagenesis: Comparison of Earth and Mars Concretions" (PDF). GSA Today. 15 (8): 4–10. doi:10.1130/1052-5173(2005)015[4:RRARPD]2.0.CO;2. Retrieved 18 August 2021.
- ^ Loope, David B.; Kettler, Richard M.; Weber, Karrie A. (September 2011). "Morphologic Clues to the Origins of Iron Oxide–Cemented Spheroids, Boxworks, and Pipelike Concretions, Navajo Sandstone of South-Central Utah, U.S.A." The Journal of Geology. 119 (5): 505–520. Bibcode:2011JG....119..505L. doi:10.1086/661110. S2CID 10139364.
- ^ Hattin, D.E. (1982). "Stratigraphy and depositional environment of the Smoky Hill Chalk Member, Niobrara Chalk (Upper Cretaceous) of the type area, western Kansas". Kansas Geological Survey Bulletin. 225: 1–108.
- ^ Hobbs, D; Hafner, J (25 October 1999). "Magnetism and magneto-structural effects in transition-metal sulphides". Journal of Physics: Condensed Matter. 11 (42): 8197–8222. Bibcode:1999JPCM...11.8197H. doi:10.1088/0953-8984/11/42/303. S2CID 250900204.
- ^ Hoffmann, Viktor; Stanjek, Helge; Murad, Enver (December 1993). "Mineralogical, magnetic and mössbauer data of symthite (Fe9S11)". Studia Geophysica & Geodætica. 37 (4): 366–381. Bibcode:1993StGG...37..366H. doi:10.1007/BF01613583. S2CID 131123088.
- ^ Gratacap, L.P. (1884). "Opinions Upon Clay Stones and Concretions". The American Naturalist. 18 (9): 882–892. doi:10.1086/273756. S2CID 84690956. Retrieved 18 August 2021.
- ^ Sheldon, J.M.A. (1900). Concretions from the Champlain clays of the Connecticut Valley. Boston: University Press. p. 74. Retrieved 18 August 2021.
- ^ Tarr, W. A. (31 October 1935). "Concretions in the Champlain formation of the Connecticut River Valley". Geological Society of America Bulletin. 46 (10): 1493–1534. Bibcode:1935GSAB...46.1493T. doi:10.1130/GSAB-46-1493.
- ^ Kindle, E. M. (30 September 1923). "Range and Distribution of Certain Types of Canadian Pleistocene Concretions". Geological Society of America Bulletin. 34 (3): 609–648. Bibcode:1923GSAB...34..609K. doi:10.1130/GSAB-34-609.
- ^ Warkentin, B.P., 1967. Carbonate content of concretions in varved sediments. Canadian Journal of Earth Sciences, 4(2), pp.333-333.
- ^ Haigney, Sophie (2021-06-18). "Once Again, Fossils Are Hot". The New York Times. ISSN 0362-4331. Retrieved 2021-07-14.
External links
[edit]- Dietrich, R.V., 2002, Carbonate Concretions – A Bibliography, The Wayback Machine. and PDF file of Carbonate Concretions – A Bibliography Archived 2014-12-17 at the Wayback Machine, CMU Online Digital Object Repository, Central Michigan University, Mount Pleasant, Michigan.
- Biek, B., 2002, Concretions and Nodules in North Dakota North Dakota Geological Survey, Bismarck, North Dakota.
- Everhart, M., 2004, A Field Guide to Fossils of the Smoky Hill ChalkPart 5: Coprolites, Pearls, Fossilized Wood and other Remains Part of the Oceans of Kansas web site.
- Hansen, M.C., 1994, Ohio Shale Concretions PDF version, 270 KB Ohio Division of Geological Survey GeoFacts n. 4, pp. 1–2.
- Hanson, W.D., and J.M. Howard, 2005, Spherical Boulders in North-Central Arkansas PDF version, 2.8 MB Arkansas Geological Commission Miscellaneous Publication n. 22, pp. 1–23.
- Heinrich, P.V., 2007, The Giant Concretions of Rock City Kansas PDF version, 836 KB Archived 2016-10-20 at the Wayback Machine BackBender's Gazette. vol. 38, no. 8, pp. 6–12.
- Hokianga Tourism Association, nd, Koutu Boulders ANY ONE FOR A GAME OF BOWLS? and Koutu Boulders, Hokianga Harbour, Northland, New Zealand High-quality pictures of cannonball concretions.
- Irna, 2006, All that nature can never do, part IV : stone spheres Archived 2016-03-03 at the Wayback Machine
- Irna, 2007a, Stone balls : in France too! Archived 2015-07-13 at the Wayback Machine
- Irna, 2007b, Stone balls in Slovakia, Czech Republic and Poland Archived 2008-03-15 at the Wayback Machine
- Katz, B., 1998, Concretions Digital West Media, Inc.
- Kuban, Glen J., 2006–2008. Nevada Shoe Prints?
- McCollum, A., nd, Sand Concretions from Imperial Valley, a collection of articles maintained by an American artist.
- Mozley, P.S., Concretions, bombs, and groundwater, on-line version of an overview paper originally published by the New Mexico Bureau of Geology and Mineral Resources.
- United States Geological Survey, nd, Cannonball concretion
- University of Utah, 2004, Earth Has 'Blueberries' Like Mars 'Moqui Marbles' Formed in Groundwater in Utah's National Parks Archived 2015-08-02 at the Wayback Machine press release about iron oxide and Martian concretions
- Tessa Koumoundouros: These Eerie 'Living Stones' in Romania Are Fantastical, And Totally Real. On: sciencealert. 25 December 2020: About Trovants in Costești, Ulmet and other locations in Romania